News
Hill Prizes Information Session
Join TAMEST for an information session on the Hill Prizes, funded by Lyda Hill Philanthropies, on Thursday, April 18, 2024, from 10 a.m.–11 a.m. CT via Zoom to learn more about the prizes, application process and selection criteria. Applications for the 2025 Hill Prizes open May 1 and close May 31, 2024.
Video and Slides from the 2025 Edith and Peter O’Donnell Awards Information Session are Now Available
Video and slides from the 2025 Edith and Peter O’Donnell Awards information session, hosted by Committee Chair Margaret A. Goodell, Ph.D. (NAM), Baylor College of Medicine, are now available. The session provided information about the nomination and selection process for the awards. Nominations are open for the 2025 Edith and Peter O’Donnell Awards through April 30, 2024.
TAMEST Member Profile: TAMEST Edith and Peter O’Donnell Awards Committee Chair Margaret “Peggy” A. Goodell, Ph.D. (NAM), Baylor College of Medicine
TAMEST Member Margaret “Peggy” A. Goodell, M.D. (NAM), Baylor College of Medicine, made TAMEST history this year as the first former Edith and Peter O’Donnell Awards Recipient to chair the O’Donnell Awards selection committee. TAMEST connected with Dr. Goodell to learn more about her innovative stem cell research and to discuss why TAMEST members should recognize the next generation of research leaders by submitting a nomination for the 2025 Edith and Peter O’Donnell Awards.
TAMEST Members News Roundup- March 2024
From award nominations to media interviews, TAMEST loves to share the accolades of our membership. Read the March compilation of our membership’s recent accomplishments, research breakthroughs and more.
TAMEST 2024 Annual Conference: Photos, Slides and Videos Available
Thank you to all who joined us at the TAMEST 2024 Annual Conference: Artificial Intelligence and Machine Learning, February 5-7, 2024, at the AT&T Hotel and Conference Center in Austin, Texas, as we celebrated 20 years of TAMEST. Speaker slides, videos and photos are now available. We’re pleased to share some highlights of the meeting with you.
2024 Hill Prizes Announced; Prize Program Extended and Expanded
TAMEST and Lyda Hill Philanthropies have announced the recipients of the 2024 Hill Prizes. The prizes, funded by Lyda Hill Philanthropies, accelerate high-risk, high-reward research ideas with significant potential for real-world impact. After an incredibly successful first year, Lyda Hill Philanthropies has committed over $10 million in funding to continue the prize program for the next three years. The $10 million will include the addition of a new prize in the category of Public Health, resulting in six prizes per year of $500,000 each.
TAMEST Welcomes Four New Members Elected to the National Academy of Engineering (NAE)
TAMEST congratulates four new members on their election to the National Academy of Engineering (NAE). Election to the National Academy of Engineering is among the highest professional distinctions afforded to an engineer. This year, the NAE elected 114 new members and 21 international members, which brought the total U.S. membership to 2,310 and the number of international members to 332. TAMEST will welcome these and other new members at the opening reception of the TAMEST 2025 Annual Conference in Irving, Texas.
TAMEST Member Profile: Karen Wooley, Ph.D. (NAS), Texas A&M University
TAMEST congratulates four new members on their election to the National Academy of Engineering (NAE). Election to the National Academy of Engineering is among the highest professional distinctions afforded to an engineer. This year, the NAE elected 114 new members and 21 international members, which brought the total U.S. membership to 2,310 and the number of international members to 332. TAMEST will welcome these and other new members at the opening reception of the TAMEST 2025 Annual Conference in Irving, Texas.
TAMEST Members News Roundup- February 2024
From award nominations to media interviews, TAMEST loves to share the accolades of our membership. Read the February compilation of our membership’s recent accomplishments, research breakthroughs and more.
Recipients of the 2024 Hill Prizes Announced
TAMEST and Lyda Hill Philanthropies are pleased to announce the recipients of the 2024 Inaugural Hill Prizes. The prizes, funded by Lyda Hill Philanthropies, accelerate high-risk, high-reward research ideas with significant potential for real-world impact. The prizes are given in five categories: Medicine, Engineering, Biological Sciences, Physical Sciences and Technology. Learn more about the 2024 Hill Prize recipients.
Nominations Now Open: 2025 Edith and Peter O’Donnell Awards
Nominations are now open for the 2025 Edith and Peter O’Donnell Awards. The awards annually recognize rising Texas researchers who are addressing the essential role that science and technology play in society, and whose work meets the highest standards of exemplary professional performance, creativity and resourcefulness. The nomination deadline is April 30, 2024.
2024 TAMEST Edith and Peter O’Donnell Awards Recipients Announced
The Edith and Peter O’Donnell Awards annually recognize rising Texas researchers who are addressing the essential role that science and technology play in society, and whose work meets the highest standards of exemplary professional performance, creativity and resourcefulness. Learn more about the 2024 Edith and Peter O’Donnell Awards recipients.
TAMEST Names Nidhi Sahni, Ph.D., as the Recipient of the 2024 Mary Beth Maddox Award & Lectureship
TAMEST has announced Nidhi Sahni, Ph.D., The University of Texas MD Anderson Cancer Center, as the recipient of the 2024 Mary Beth Maddox Award and Lectureship in cancer research. She was chosen for her role in identifying novel biomarkers and drug targets, which are expected to have a significant impact on cancer by translating into more effective prognosis and therapy for the disease.
TAMEST Member Profile: TAMEST 2024 Annual Conference Co-Chair Karen E. Willcox, Ph.D. (NAE), The University of Texas at Austin
TAMEST Member and TAMEST 2024 Annual Conference Co-Chair Karen E. Willcox, Ph.D. (NAE), is the Director of the Oden Institute for Computational Engineering and Sciences, Associate Vice President for Research and Professor of Aerospace Engineering and Engineering Mechanics at The University of Texas at Austin. TAMEST connected with Dr. Willcox to learn more about her work, leadership of the Oden Institute in its 50th year and helping to shape the TAMEST 2024 Annual Conference: Artificial Intelligence and Machine Learning taking place in Austin next month.
TAMEST Members News Roundup- January 2024
From award nominations to media interviews, TAMEST loves to share the accolades of our membership. Read the January compilation of our membership’s recent accomplishments, research breakthroughs and more.
TAMEST Member Profile: Lisa T. Su, Ph.D. (NAE), Chair and CEO, AMD
As Chair and Chief Executive Officer of AMD, TAMEST Member Lisa T. Su, Ph.D. (NAE), has helped transform one of the fastest growing semiconductor and adaptive computing companies in the world. TAMEST connected with Dr. Su to learn more about her passion for mentorship and powering the products and services that help solve the world’s most important challenges, and to discuss what AMD is doing to transform the semiconductor industry with artificial intelligence.
TAMEST Recognizes CPRIT with Kay Bailey Hutchison Distinguished Service Award
TAMEST has announced the Cancer Prevention and Research Institute of Texas (CPRIT) as the recipient of the Kay Bailey Hutchison Distinguished Service Award. TAMEST is recognizing CPRIT for their work improving the lives of Texans, advancing cancer research and prevention, and recruiting National Academy members to Texas. CPRIT’s success in attracting top-tier cancer scientists and companies to the state is an enormous benefit to Texas. It has catalyzed collaboration and connected research universities, doctors, scientists, hospitals, and companies across the state to form a cancer-fighting powerhouse.
TAMEST Protégé Program Continues to Lift Up the Next Generation of Researchers in Texas
TAMEST members have a unique opportunity each year to nurture the next generation of researchers in Texas. Through the TAMEST Protégé Program, early-career researchers are nominated on an individual basis by TAMEST members to attend the TAMEST annual conference at the special rate of $75. TAMEST also hosts a Protégé Poster Challenge which provides protégés an opportunity to showcase their research and innovation to research and industry leaders at the conference.
TAMEST Members News Roundup- December 2023
From award nominations to media interviews, TAMEST loves to share the accolades of our membership. Read the December compilation of our membership’s recent accomplishments, research breakthroughs and more.
TAMEST Board of Directors Meets with UT Austin Leadership
The TAMEST Board of Directors met with The University of Texas at Austin leadership in September to discuss UT Austin’s strategic plan and expanding research initiatives across the institution. Guests included UT Austin President Jay C. Hartzell, Ph.D.; Vice President for Research Daniel T. Jaffe, Ph.D.; and Executive Vice President and Provost and TAMEST Member Sharon L. Wood, Ph.D. (NAE).
Three Texans Elected to the National Academy of Medicine at Annual Meeting
TAMEST is proud to welcome three new members, including a past TAMEST Edith and Peter O’Donnell Award recipient, elected to the National Academy of Medicine (NAM) in 2023. The three were among the 90 members and 10 international members elected who have demonstrated outstanding professional achievement and commitment to service.
TAMEST Staff Profile: Crystal Tucker, Information Systems Manager
Crystal Tucker, has been with the organization for more than 15 years. Starting in 2008 as an Administrative Associate, she now serves as its Information Systems Manager and works behind the scenes to ensure TAMEST’s website and databases run smoothly. TAMEST connected with Crystal to learn more about her work for TAMEST and how its mission has inspired her for the past 15 years.
TAMEST Members News Roundup- October 2023
From award nominations to media interviews, TAMEST loves to share the accolades of our membership. Read the October compilation of our membership’s recent accomplishments, research breakthroughs and more.
Registration Open: TAMEST 2024 Annual Conference: Artificial Intelligence and Machine Learning
Registration is open for the TAMEST 2024 Annual Conference: Artificial Intelligence and Machine Learning, February 5–7, 2024, at the AT&T Hotel and Conference Center in Austin, Texas. Join us as we celebrate 20 years of TAMEST advancing research in Texas. The conference will explore the current practical and clinical use of AI/ML, examine its limitations and investigate its true potential for significant advancements in science and society.
2023 Mary Beth Maddox Awardee Featured in MD Anderson Blaffer Lecture Series
Mary Beth Maddox Award and Lectureship Recipient Florencia McAllister, M.D., MD Anderson Cancer Center, gave a presentation on her groundbreaking research on September 12, 2023 to more than 60 individuals as part of MD Anderson Cancer Center’s John H. Blaffer Lecture Series. Dr. McAllister presented her lecture, Microbial Matters in Pancreatic Cancer, on her seminal discoveries at the intersection of microbes, the immune system and pancreatic cancer.
TAMEST Member Profile: Katherine Freese, Ph.D. (NAS), The University of Texas at Austin
TAMEST Member Katherine Freese, Ph.D. (NAS), The University of Texas at Austin, is a world-renowned theoretical astrophysicist who recently discovered a possible new type of star, powered by dark matter itself. TAMEST spoke with Dr. Freese about what this recent discovery could mean for our understanding of the universe and why early mentorships are so vital in the trajectory of rising researchers.
TAMEST Members News Roundup- September 2023
From award nominations to media interviews, TAMEST loves to share the accolades of our membership. Read the September compilation of our membership’s recent accomplishments, research breakthroughs and more.
TAMEST Mourns the Loss of Beloved TAMEST Member and Former Board President J. Tinsley Oden, Ph.D. (NAE), The University of Texas at Austin
TAMEST mourns the loss of beloved TAMEST Member and Former Board President J. Tinsley Oden, Ph.D. (NAE), The University of Texas at Austin. Dr. J. Tinsley Oden, was widely known as the founder of computational mechanics and the first director of what is now known as the Oden Institute for Computational Engineering and Sciences at The University of Texas at Austin.
Mary Beth Maddox Award and Lectureship Information Session Recording and Slides Now Available
On August 24, TAMEST hosted an information session on the Mary Beth Maddox Award and Lectureship to share more about the program and nomination process. Video and slides from the session are now available. Nominations for the 2024 Mary Beth Maddox Award and Lectureship are currently open and will close on September 25, 2023.
2023 Mary Beth Maddox Award Recipient to Give Lecture at MD Anderson
Join TAMEST and 2023 Mary Beth Maddox Award and Lectureship Recipient Florencia McAllister, M.D., MD Anderson Cancer Center, for a presentation on her groundbreaking cancer research hosted by MD Anderson Cancer Center’s John H. Blaffer Lecture Series. The lecture will take place on Tuesday, September 12, 2023, from 12 p.m. to 1 p.m. at MD Anderson’s Onstead Auditorium (6767 Bertner Ave.) in Houston. No RSVP is required to attend.
TAMEST Member Profile: Helen Heslop, M.D., D.Sc. (NAM), Baylor College of Medicine; TAMEST Mary Beth Maddox Award and Lectureship Committee Member
TAMEST Member Helen Heslop, M.D., D.Sc. (NAM), Baylor College of Medicine, is a physician-scientist engaged in translational research which aims to improve hemopoietic stem cell transplantation and cancer therapy. TAMEST connected with Dr. Heslop to learn more about her work guiding cutting-edge cancer research and gene therapy in the state of Texas and her new role on the TAMEST Mary Beth Maddox Award and Lectureship Committee.
TAMEST Members News Roundup- August 2023
From award nominations to media interviews, TAMEST loves to share the accolades of our membership. Read the August compilation of our membership’s recent accomplishments, research breakthroughs and more.
Hill Prizes Information Session Recording and Slides Now Available
On July 12, TAMEST hosted an information session on the new Hill Prizes, funded by Lyda Hill Philanthropies. More than 200 individuals joined the session to learn more about the program, application process and selection criteria. Video and slides from the session are now available. The deadline to apply for a Hill Prize is August 11, 2023.
Mary Beth Maddox Award and Lectureship Nominations Open August 1
Nominations for the 2024 Mary Beth Maddox Award and Lectureship will open August 1, 2023 through September 25, 2023. The award recognizes women scientists in Texas bringing new ideas and innovations to the fight against cancer. The recipient will be recognized with a $5,000 honorarium and award and present their research at the TAMEST 2024 Annual Conference in Austin, Texas.
TAMEST Profile: Joshua T. Mendell, M.D., Ph.D., UT Southwestern Medical Center; 2023 TAMEST Annual Conference Co-Chair; 2016 Edith and Peter O’Donnell Award Recipient
Dr. Joshua T. Mendell, UT Southwestern Medical Center, knew from an early age that he wanted to pursue a career in research. The son of a physician–scientist, he began working in a molecular biology laboratory when he was just a teenager and continued to perform laboratory research as an undergraduate at Cornell University. TAMEST connected with Dr. Mendell to hear more about his research and why it is so important to support the next generation of research leaders.
TAMEST Members News Roundup- July 2023
From award nominations to media interviews, TAMEST loves to share the accolades of our membership. Read the July compilation of our membership’s recent accomplishments, research breakthroughs and more.
TAMEST 2023 Annual Conference: Slides and Photos Available
The TAMEST 2023 Annual Conference: Forward Texas — Accelerating Change gathered some of our most celebrated and innovative researchers to examine how science and technology are leading to fundamental changes in society. Speaker slides and photos are now available from the May 23–25 conference sessions.
TAMEST Profile: Maksim Mamonkin, Ph.D., Baylor College of Medicine, TAMEST 2023 Protégé Poster Challenge Winner
TAMEST 2023 Protégé Poster Challenge Winner Maksim Mamonkin, Ph.D., Baylor College of Medicine, first came to Texas in 2007 from Russia as a graduate student studying immunology. Now, he is an Associate Professor of Pathology and Immunology at the Center for Cell and Gene Therapy at Baylor College of Medicine, where he is known for bringing his creative thinking and deep immunology skills to the field of cell therapy and cancer treatment. TAMEST connected with Dr. Mamonkin to learn more about his cancer research and decision to participate in TAMEST’s protégé program.
TAMEST and Lyda Hill Philanthropies Announce the Hill Prizes
Texas scientists will receive $2.5 million in funding to advance their research thanks to a new prize program from Lyda Hill Philanthropies and TAMEST. The Hill Prizes, funded by Lyda Hill Philanthropies, will accelerate high-risk, high-reward research ideas with significant potential for real-world impact.
TAMEST Welcomes Five Texans Elected to the National Academy of Sciences in 2023
TAMEST is proud to welcome three new members and two current members elected to the National Academy of Sciences (NAS) in 2023. The five were among the 120 members and 23 international members elected in recognition of their distinguished and continuing achievements in original research.
TAMEST Members News Roundup- June 2023
From award nominations to media interviews, TAMEST loves to share the accolades of our membership. Read the June compilation of our membership’s recent accomplishments, research breakthroughs and more.
Happening Next Month: TAMEST 2023 Annual Conference
Join us for the TAMEST 2023 Annual Conference: Forward Texas — Accelerating Change, May 23–25, 2023, at The InterContinental — Medical Center in Houston, Texas. The conference will gather some of our most celebrated and innovative researchers to examine how science and technology are leading to fundamental changes in society. The TAMEST Annual Conference is open to the entire research community, including scientists at all levels of training.
2024 Edith and Peter O’Donnell Awards Call for Nominations
This is the last chance to nominate for the 2024 Edith and Peter O’Donnell Awards. Don’t miss this opportunity to recognize the outstanding achievements of a rising star researcher. The 2024 O’Donnell Awards will recognize recipients in the categories of Medicine, Engineering, Biological Sciences, Physical Sciences and Technology Innovation. The nomination deadline is April 30, 2023.
TAMEST Member Profile: Karen Lozano, Ph.D. (NAE), The University of Texas Rio Grande Valley
TAMEST Member Karen Lozano, Ph.D. (NAE) made history this year as the first-ever faculty member from The University of Texas Rio Grande Valley to be elected to the National Academy of Engineering for contributions to nanofiber research and commercialization. TAMEST connected with Dr. Lozano to hear more about her passion for nanotechnology, research and mentoring undergraduate students from underserved populations.
TAMEST Members News Roundup- April 2023
From award nominations to media interviews, TAMEST loves to share the accolades of our membership. Read the April compilation of our membership’s recent accomplishments, research breakthroughs and more.
TAMEST Names MD Anderson’s Dr. Florencia McAllister as the Recipient of the 2023 Mary Beth Maddox Award & Lectureship
TAMEST is pleased to announce Florencia McAllister, M.D., The University of Texas MD Anderson Cancer Center, as the recipient of the 2023 Mary Beth Maddox Award and Lectureship in cancer research. She was chosen for her seminal discoveries at the intersection of microbes, the immune system and pancreatic cancer, leading to insights into early detection, prevention and therapeutic strategies to fight the disease.
TAMEST Welcomes Six New Member Institutions in 2023
TAMEST is proud to welcome six new member institutions in 2023. The new institutions include Baylor University, Texas Tech University Health Sciences Center, The University of North Texas Health Science Center, The University of Texas at El Paso, The University of Texas Medical Branch at Galveston and The University of Texas Rio Grande Valley.
ACM Turing Award Honors Bob Metcalfe for Ethernet Invention
ACM, the Association for Computing Machinery, named TAMEST Member Bob Metcalfe, Ph.D. (NAE), The University of Texas at Austin, as recipient of the 2022 ACM A.M. Turing Award for the invention, standardization, and commercialization of Ethernet. The ACM A.M. Turing Award, often referred to as the “Nobel Prize of Computing,” carries a $1 million prize with financial support provided by Google, Inc.
TAMEST Staff Profile: Stephanie Shaw, TAMEST Program Officer
As TAMEST’s Program Officer, Stephanie Shaw is responsible for managing all TAMEST programs, including the Edith and Peter O’Donnell Awards, Mary Beth Maddox Award and Lectureship and the TAMEST Protégé Program. TAMEST connected with Stephanie to learn more about her passion for her work and to hear more about the latest in TAMEST programs.
TAMEST Members News Roundup- March 2023
From award nominations to media interviews, TAMEST loves to share the accolades of our membership. Read the March compilation of our membership’s recent accomplishments, research breakthroughs and more.
TAMEST Welcomes Four New Members Elected to the National Academy of Engineering (NAE)
TAMEST congratulates four new members on their election to the National Academy of Engineering (NAE). The group includes the first faculty member from The University of Texas Rio Grande Valley (UTRGV) to be elected to a National Academy. New TAMEST Member Karen Lozano, Ph.D. (NAE), UTRGV, was also a speaker at one of TAMEST’s first research summits in 2007.
Nominations are Now Open for the 2024 Edith and Peter O’Donnell Awards
Nominations are now open for the 2024 Edith and Peter O’Donnell Awards. The awards annually recognize rising Texas researchers who are addressing the essential role that science and technology play in society, and whose work meets the highest standards of exemplary professional performance, creativity and resourcefulness. Nominations for the 2024 O’Donnell Awards are open February 1, 2023 – April 30, 2023.
TAMEST to Host Information Sessions on O’Donnell Award Nominations
An informational session on the Edith and Peter O’Donnell Awards will take place on Tuesday, March 7, at 11:00–11:45 a.m. CST for the Medicine, Engineering, Biological Sciences & Physical Sciences categories and at 2:00–2:45 p.m. CST for the Technology Innovation category. Registration is required and attendees will have an opportunity to ask questions during the session.
TAMEST Member Profile: Elaine Oran, Ph.D. (NAE), Texas A&M University
TAMEST Member Elaine Oran, Ph.D. (NAE), Texas A&M University (TAMU), was recruited to Texas from Maryland in 2019 as a Professor of Aerospace Engineering and O’Donnell Foundation Chair IV, with the promise of creating an enormous explosion testing facility on TAMU’s RELLIS campus. TAMEST connected with Dr. Oran to learn how her methods unify concepts from science, physics, mathematics, engineering and computer science to form a new methodology.
TAMEST Members News Roundup- February 2023
From award nominations to media interviews, TAMEST loves to share the accolades of our membership. Read the February compilation of our membership’s recent accomplishments, research breakthroughs and more.
2023 TAMEST Edith and Peter O’Donnell Awards Recipients Announced
The Edith and Peter O’Donnell Awards annually recognize rising Texas researchers who are addressing the essential role that science and technology play in society, and whose work meets the highest standards of exemplary professional performance, creativity and resourcefulness. Learn more about the 2023 Edith and Peter O’Donnell Awards recipients.
TAMEST Board Appoints New Leadership
At its January 24, 2023 meeting, the TAMEST Board of Directors officially appointed Brendan Lee, M.D., Ph.D. (NAM), Baylor College of Medicine as President and Ganesh Thakur, Ph.D. (NAE), University of Houston as Vice President. Both will serve a two-year term. The TAMEST Board President and Vice President help coordinate and guide the TAMEST Board of Directors in planning strategy, programs and communications.
TAMEST Video Profile: TAMEST Board President Brendan Lee, M.D., Ph.D. (NAM), Baylor College of Medicine
TAMEST connected with Board President Brendan Lee, M.D., Ph.D. (NAM), Baylor College of Medicine, to learn more about his dedication to TAMEST and what he sees in the organization’s future.
TAMEST Members News Roundup- January 2023
From award nominations to media interviews, TAMEST loves to share the accolades of our membership. Read the January compilation of our membership’s recent accomplishments, research breakthroughs and more.
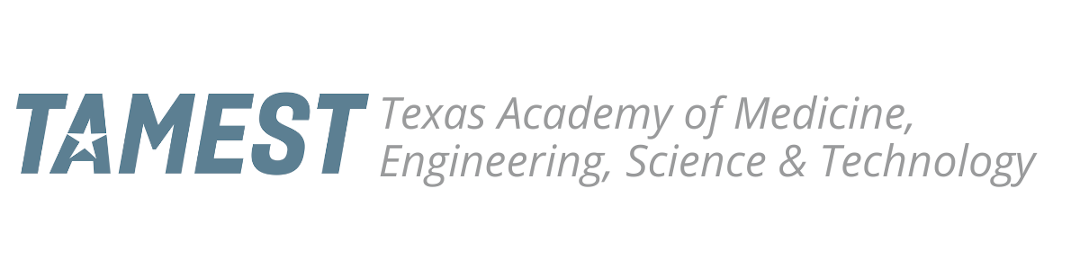