TAMEST Member Profile: Karen Wooley, Ph.D. (NAS), Texas A&M University
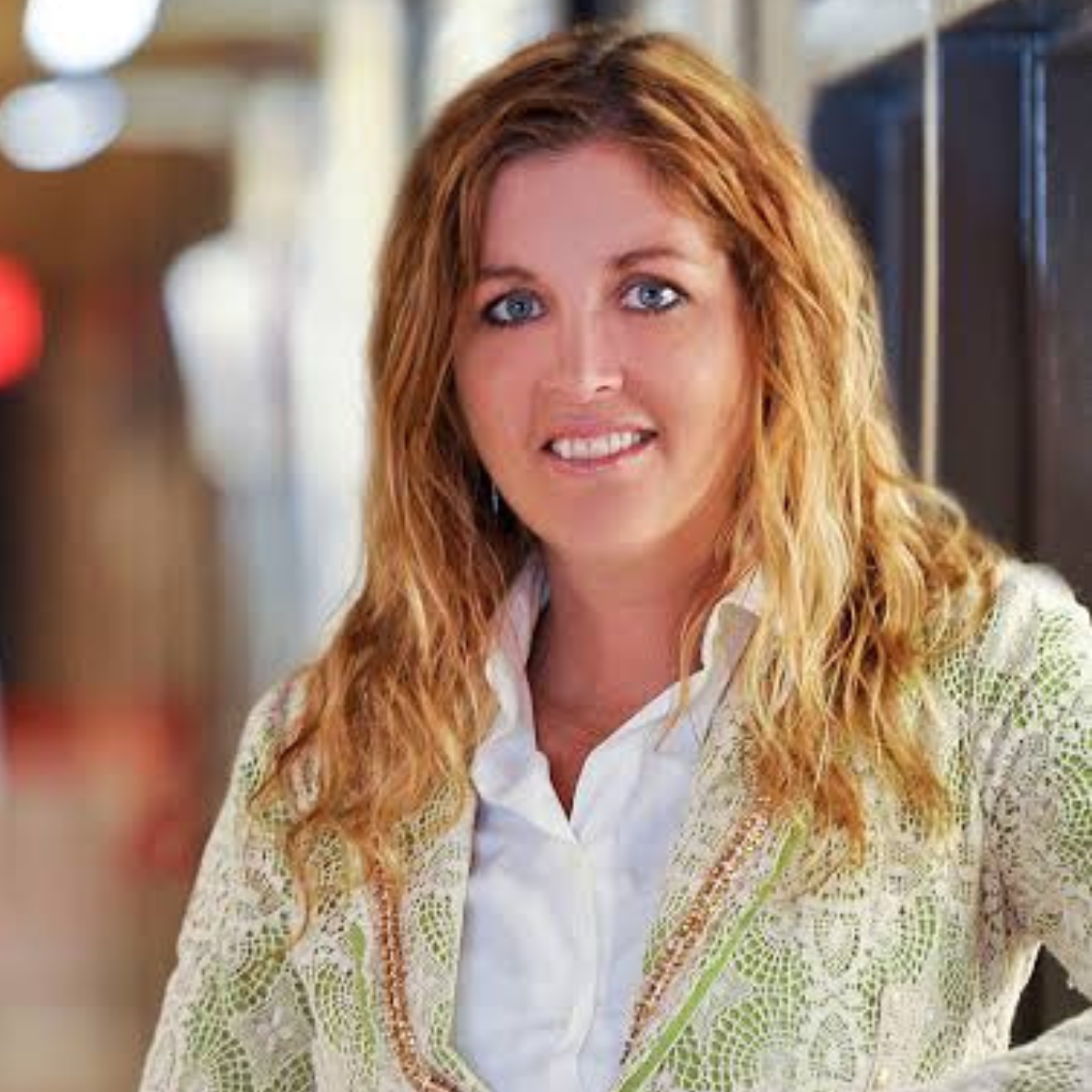
TAMEST Member Karen Wooley, Ph.D. (NAS), Texas A&M University, is one of the world’s top chemists in the burgeoning field of materials and polymer chemistry and in creating new materials at the nanoscale level.
She is a Distinguished Professor and W.T. Doherty-Welch Foundation Chair at Texas A&M and the Director of the TAMU Laboratory for Synthetic-Biologic Interactions. Her research interests are focused broadly upon the design, synthesis and characterization of unique polymers, with an emphasis on the development of synthetic methodologies that allow for the preparation of complex nanostructured materials.
With a keen eye for societal and environment impact, Dr. Wooley’s work aims to identify natural products to create plastics that are sustainable, both in their end-of-life by being made to be degradable and digestible, and in their start-of-life by originating from feedstocks that do not compete with food sources like plants and trees.
The building blocks that she transforms into polymers that behave as plastics include sugars, amino acids, lipids and other small molecules, and the structures for those polymers range from macromolecular to nanoscopic (like proteins, lipoproteins, viral capsids and other biological materials). She has helped to pioneer metal-free, recyclable polypeptide batteries, utilized dead flies to create biodegradable plastics and more.
TAMEST connected with Dr. Wooley to learn more about how she utilizes her specialized skillset and research for a more sustainable future while also helping to train the next generation of chemist leaders at Texas A&M.
Please tell us a little about yourself and how you found your career path at the intersection of chemistry, chemical engineering and synthetic biology.
It really came down to the fact that I always enjoyed chemistry. I had two years of chemistry in high school and when I was trying to decide what I wanted to study in college I just thought chemistry is something that I enjoyed and was pretty good at it. So, I decided to try that. I got involved in research my freshman year at Oregon State University in a synthetic organic chemistry lab making small biological molecules.
My first interest was the ability to control matter and experience the frontier of making something that no one else had ever made or studied. I found it fascinating. Then it evolved when I went to graduate school at Cornell University and started studying polymers, which had fascinated me my last few years as an undergraduate. I loved the idea of moving beyond the small molecules that I’d been synthesizing for a biological response to something that I could hold in my hands and stretch. I liked understanding the mechanical properties, the thermal properties and all the characteristics that could make them useful in society. I mean, plastics are everywhere.
I studied dendritic (tree-like) macromolecules for my Ph.D. There I was working on this really interesting project where we were expanding beyond linear polymer chains. It was this curiosity driven research. We didn’t really know what they might be useful for or the real-life societal applications, but it was the challenge of the synthesis. When I started my own independent career as an Assistant Professor at Washington University in St. Louis, I wanted to make big versions of three-dimensionally well-defined macromolecules in a fast, easy way. That led us to a method to build nanoparticles, not from small molecules directly, but rather by a combination of pre-construction of amphiphilic block copolymers followed by their supramolecular assembly and covalent stabilization. This was all when nanoparticles were just emerging. I kept thinking that we’d developed synthetic methods to make these nanoparticles and they had these interesting properties, but what can we do with them?
So, it is a long story, but that’s what took me into these other disciplines; into engineering and drug delivery, into my interactions with biological systems, all of it. It expanded my primary role as a synthetic chemist into the other disciplines. It was this long pathway over a couple of decades, but it has been rewarding every single day. There are so many different directions to take.
You work a lot with polymers and organic molecules to organize larger composites that fold into intricate and useful shapes of soft materials. What makes you most passionate about your work?
Sometimes it’s just curiosity and the intellectual challenge. I love seeing if we can develop synthetic methods that will let us make materials that haven’t been made before. Other times we see a need – an environmental need or societal need – and we think of how we can design material that can address it.
My passion comes both ways: curiosity and impact. I’ll give you one example. Over the last 10 to 15 years, we’ve been successful in designing synthetic methods which take sugars and build them into well-defined polymers. There are a lot of polysaccharides in nature but the way we connect the sugars together includes linkages that can be broken under certain conditions. So, we’re building in this end of life into polymers of sugars. Initially, we wanted to make some materials that might be useful for orthopedic applications in bone repair, but it’s turned into this huge academic initiative here at Texas A&M and also commercial translation through a couple of startup companies that have spun out of it.
One of the startups is called Sugar Plastics, LLC and the other one is Teysha Technologies, Ltd. Sugar Plastics is exploring things like sports fishing equipment, with an initial emphasis on soft plastic fishing lures. If they’re lost in the environment or ingested by either a fish or other wildlife, they can be digested and/or degraded and broken down. Teysha has a focus on addressing plastics pollution, for instance that derives from plastic packaging, and are also working on other things like biodegradable chewing gum base. The possibilities are just endless and that makes me very passionate.
Recently, your research showed how dead flies could be used to make biodegradable plastic. Talk about this finding and what it could mean for the future of polymers and plastics.
I’ve mentioned sugar several times. Originally, we were designing our materials based on cellulose, which is a polymer of glucose. We wanted to take the glucose units that are present in cellulose and connect them, not through what’s called a glycosidic linkage but rather through a carbonate linkage that would then allow for hydrolysis to break down the polymer to its building blocks of glucose and carbon dioxide, and it wouldn’t require cellulase enzymes to do so. We started considering getting our glucose from cellulose, which is the predominant structural material found in plants. Glucose is also the building block of starch. So, we’re taking plants and then breaking them down into glucose or other sugars and then build them up into plastics.
Then, one of my colleagues, Dr. Jeff Tomberlin, who’s in the Department of Entomology at Texas A&M, mentioned to me that he has this program where he’s growing black soldier flies as an agricultural crop. Black soldier flies, mealworms and other insects are grown not only as an agricultural crop, where the larvae serve as feed for animals like for pig farms and fish hatcheries, but also in other parts of the world as food for humans.
The adult flies basically reproduce, lay eggs and die. So, he asked me if we could do something to utilize this waste of dead adult black solider fly carcasses. I said of course we can! We can extract from them various components, like lipids and chitin. One of their major components is chitin, which is found in shrimp shells and crab shells, too. It’s being harvested as a material either to be used as chitin or as chitosan, after some degree of deacetylation.
When I started thinking about this, I thought we could try something. In fact, the real impact isn’t just that we’ll take this waste and turn it into bioplastic, but it means we don’t have to take plants to get our bioplastics. If we don’t have to use plants to get our bioplastics, then we’re not competing with those plants for being a food source or a fuel source. The impact of this might be the greatest in the fact that we’re diverting away from competing with other societal needs where we harvest our feedstocks to make the plastic materials.
Previously, you’ve also worked with colleagues to develop new metal-free, recyclable polypeptide batteries that degrade on demand. Why is focusing on batteries so important?
This came from discussions with my colleague, TAMEST 2022 Edith and Peter O’Donnell Award Recipient in Engineering, Dr. Jodie Lutkenhaus. She and I began talking because metal-free organic polymer battery research is her baby and most of her organic radical polymer materials are based on petrochemical feed stocks. One of my interests, aside from not using plants, is wanting to shift away from petrochemicals and use sustainable feed stocks in the making of materials. Hers were petrochemically sourced and they were non-degradable.
She and I started talking about several reasons we wanted to work in this area: one being degradability. There’s biodegradability, which requires a biological organism or an enzyme to break it down. However, there’s also other mechanisms for degradability, which could be hydrolysis involving bond cleavage by reactions water, or by other stimuli, including heat or light. We wanted to make these materials that could be either biodegradable or degradable, or even just recycled. Recycling batteries is really important, especially as we’re getting more and more batteries from electric vehicles and other elements.
Organic materials have the potential to create batteries that are nontoxic or less toxic than metal-based batteries, enhance safety and also recyclability or degradability. Ideally, if the battery is discarded then it could degrade without requiring human intervention and then it wouldn’t persist in the environment for long periods of time. In Dr. Lutkenhaus’ case, one of her key interests is getting away from metals and the way that those metals are mined, in some cases with child labor. There’s this whole societal impact. There’s an economic impact. There’s also national security that could be realized with being able to use naturally-sourced organic materials. So, Dr. Lutkenhaus brought a lot of the ideas behind the work and I brought my interest in using sustainable building blocks to make those materials that can break back down batteries to regenerate the safe, non-toxic, sustainable components from which they were initially built.
The concept has so much promise for those materials. Our first paper on it had a backbone based on amino acids, but also included redox-active side chain moieties that provided for the battery performance, however, they weren’t sustainably sourced and weren’t necessarily non-toxic. So, I have two students right now who are focused on replacing those materials with naturally sourced alternatives and getting to that next level of degradability, recyclability, safety and non-toxicity. It is very exciting.
How long until commercial biodegradable batteries are in our future?
I don’t think it’s going to take that long. To happen, it will need some industrial involvement to follow through with the research we’re doing academically.
I recently received an email from someone at a company who is looking to do exactly that. They are working on principal battery materials and want them to be recyclable and degradable. They were using our Nature publication on biodegradable batteries as a model for moving forward.
One of the things that we do in academia is we put our ideas out there. Whatever progress we make is for other people to take and run with. I’m excited to see that happening in this case.
How important is mentoring the next generation of research leaders to you?
Mentoring the next generation of research leaders is critical. I’ve talked a lot about my research and making an impact on society but really the foundational core of what I do is training the next generation of great chemists.
I do that through formal courses but really the most in-depth training that I provide is through research experiences for undergraduates, graduate students and postdoctoral associates. They are the products that come out of the university and what they do in the future amplifies us in so many different ways.
When I first started out as an Assistant Professor, I sometimes would feel that students take so long to learn something and do something that I could do much faster. But what I realized is that I have to give them the time to learn and grow and develop. They need time to become independent scientists because, ultimately, when they emerge out of training, they can do so much more than I ever could. A lot of my students have gone into industrial positions and they’re at companies having huge impacts. Some have gone into academia and are teaching the next generations as well.
I love getting surprised by emails from former students. Just over the last year or so I’ve been contacted by former undergraduate students from Washington University who I taught Organic Chemistry to decades ago who are now medical doctors, faculty or following other career paths. They’re thanking me for teaching them years and years ago and that’s so rewarding for me, just knowing that my students are out there having an impact. I wouldn’t be doing what I do if it weren’t enjoyable and rewarding.
Why do you live and work in Texas?
I really love Texas. I grew up in Oregon, went to school in upstate New York and then I lived in St. Louis for 16 years before moving to Texas. Some people say Texas is its own country. It really is a place where people care about each other and it just feels like home.
That is the case with Texas and especially with Texas A&M. The university is what brought me here and I’m still fascinated by the daily energy and enthusiasm. Our students work really hard. They are dedicated to their studies. They don’t complain. They just get in there and get the job done. They’re always striving to learn more and do better. It’s inspiring.
I get approached about jobs outside of Texas asking if I would consider moving somewhere else and I don’t even entertain those types of inquiries. At this point, I just love being in Texas, in College Station and at Texas A&M.
What does being a member of the National Academies mean to you?
It’s difficult to describe. Being a member of the National Academy of Sciences (NAS) is something I always wanted. I think almost every scientist does.
However, when I was elected to become a member, I realized it’s not just an honor but it’s an obligation. I feel obligated to do everything I can to support the whole infrastructure of science and to support my colleagues. I’ve always been supportive of junior colleagues. Now, being in NAS, it gives that extra level of responsibility and obligation. The National Academies are not merely an honor that you can sit back and enjoy. It really is a call to action to do more.
What do you enjoy doing outside of your research?
I have three sons, three dogs and 10 acres. I love hunkering down with the family and I love to camp. Recently, my family came back from a bit of time off with our fifth wheel trailer. We took it down on the Gulf between Freeport and Galveston. I love to camp and be with my boys. Well, they’re men now.
In addition, I am passionate about growing hot peppers. I grow a variety of different kinds, and then I collect the crops and turn them into relishes and all kinds of canned forms of hot peppers.
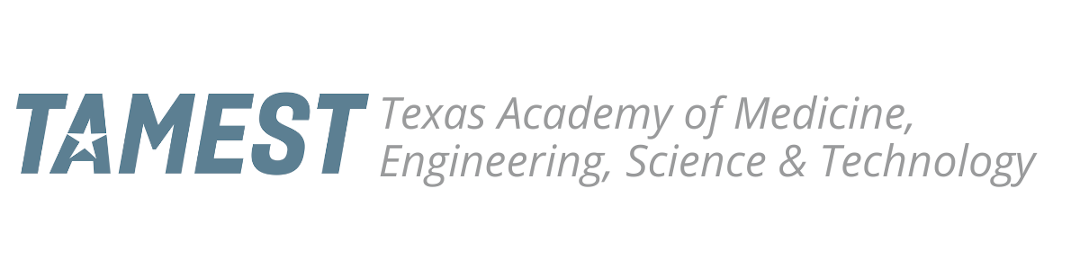